In chemistry and physics, a valence electron is an outer shell electron that is associated with an atom, and that can participate in the formation of a chemical bond if the outer shell is not closed; in a single covalent bond, both atoms in the bond contribute one valence electron in order to form a shared pair. Barium (Ba) has two valence electrons, and these electrons are located in the 6s subshell. Without using the periodic table, in which group and period is barium located? How many valence electrons does tin (Sn) have? What do elements in the p-block have in common? Valence Electrons. The electrons in the outermost shell are the valence electrons the electrons on an atom that can be gained or lost in a chemical reaction. Since filled d or f subshells are seldom disturbed in a chemical reaction, we can define valence electrons as follows: The electrons on an atom that are not present in the previous rare gas, ignoring filled d or f subshells. Barium is in Period 6, which means that in writing the configuration you should only need 6 numbers. The correct config. Should in fact be 2, 8, 18, 18, 8, 2 which means that barium has 2 valance.
- Valence Electrons In Each Element
- Be Valence Electrons Octet
- Ba Number Of Valence Electrons
- Be Valence Electrons Number
- Be Valence Electrons
Valence Electrons | The Covalent Bond | How Sharing Electrons Bonds Atoms | Similarities and Differences Between Ionic and Covalent Compounds |
Electronegativity | Using Electronegativity to Identify Ionic/Covalent/Polar Covalent Compounds | Limitations of the Electronegativity Concept | The Difference Between Polar Bonds and Polar Molecules |
The electrons in the outermost shell are the valence electronsthe electrons on an atom that can be gained or lost in a chemical reaction. Since filled d or f subshells are seldom disturbed in a chemical reaction, we can define valence electrons as follows: The electrons on an atom that are not present in the previous rare gas, ignoring filled d or f subshells.
Gallium has the following electron configuration.
Ga: [Ar] 4s23d104p1
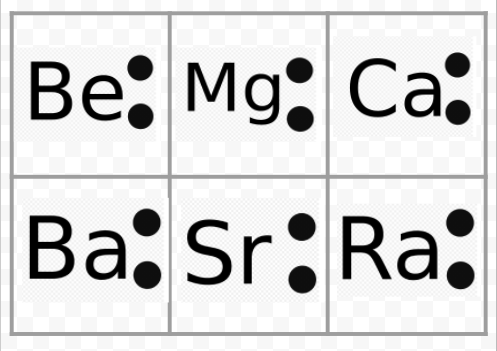
The 4s and 4p electrons can be lost in a chemical reaction, but not the electrons in the filled 3d subshell. Gallium therefore has three valence electrons.
Practice Problem 1: Determine the number of valence electrons in neutral atoms of the following elements: (a) Si (b) Mn (c) Sb (d) Pb |
Atoms can combine to achieve an octet of valence electrons by sharing electrons. Two fluorine atoms, for example, can form a stable F2 molecule in which each atom has an octet of valence electrons by sharing a pair of electrons.
A pair of oxygen atoms can form an O2 molecule in which each atom has a total of eight valence electrons by sharing two pairs of electrons.
The term covalent bond is used to describe the bonds in compounds that result from the sharing of one or more pairs of electrons.
To understand how sharing a pair of electrons can hold atoms together, let's look at the simplest covalent bondthe bond that forms when two isolated hydrogen atoms come together to form an H2 molecule.
H + H H-H
An isolated hydrogen atom contains one proton and one electron held together by the force of attraction between oppositely charged particles. The magnitude of this force is equal to the product of the charge on the electron (qe) times the charge on the proton (qp) divided by the square of the distance between these particles (r2).
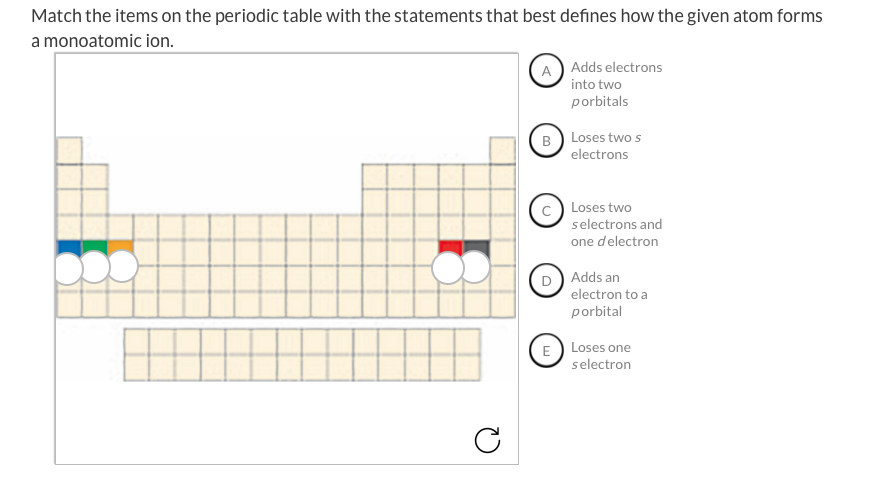
When a pair of isolated hydrogen atoms are brought together, two new forces of attraction appear because of the attraction between the electron on one atom and the proton on the other.
But two forces of repulsion are also created because the two negatively charged electrons repel each other, as do the two positively charged protons.
It might seem that the two new repulsive forces would balance the two new attractive forces. If this happened, the H2 molecule would be no more stable than a pair of isolated hydrogen atoms. But there are ways in which the forces of repulsion can be minimized. As we have seen, electrons behave as if they were tops spinning on an axis. Just as there are two ways in which a top can spin, there are two possible states for the spin of an electron: s = +1/2 and s = -1/2. When electrons are paired so that they have opposite spins, the force of repulsion between these electrons is minimized.
The force of repulsion between the protons can be minimized by placing the pair of electrons between the two nuclei. The distance between the electron on one atom and the nucleus of the other is now smaller than the distance between the two nuclei. As a result, the force of attraction between each electron and the nucleus of the other atom is larger than the force of repulsion between the two nuclei, as long as the nuclei are not brought too close together.
The net result of pairing the electrons and placing them between the two nuclei is a system that is more stable than a pair of isolated atoms if the nuclei are close enough together to share the pair of electrons, but not so close that repulsion between the nuclei becomes too large. The hydrogen atoms in an H2 molecule are therefore held together (or bonded) by the sharing of a pair of electrons and this bond is the strongest when the distance between the two nuclei is about 0.074 nm.
There is a significant difference between the physical properties of NaCl and Cl2, as shown in the table below, which results from the difference between the ionic bonds in NaCl and the covalent bonds in Cl2.
Some Physical Properties of NaCl and Cl2
NaCl | Cl2 | |
Phase at room temperature | Solid | Gas |
Density | 2.165 g/cm3 | 0.003214 g/cm3 |
Melting point | 801C | -100.98C |
Boiling point | 1413C | -34.6C |
Ability of aqueous solution to conduct electricity | Conducts | Does not conduct |
Each Na+ ion in NaCl is surrounded by six Cl- ions, and vice versa, as shown in the figure below. Removing an ion from this compound therefore involves breaking at least six bonds. Some of these bonds would have to be broken to melt NaCl, and they would all have to be broken to boil this compound. As a result, ionic compounds such as NaCl tend to have high melting points and boiling points. Ionic compounds are therefore solids at room temperature.
Cl2 consists of molecules in which one atom is tightly bound to another, as shown in the figure above. The covalent bonds within these molecules are at least as strong as an ionic bond, but we don't have to break these covalent bonds to separate one Cl2 molecule from another. As a result, it is much easier to melt Cl2 to form a liquid or boil it to form a gas, and Cl2 is a gas at room temperature.
The difference between ionic and covalent bonds also explains why aqueous solutions of ionic compounds conduct electricity, while aqueous solutions of covalent compounds do not. When a salt dissolves in water, the ions are released into solution.
These ions can flow through the solution, producing an electric current that completes the circuit. When a covalent compound dissolves in water, neutral molecules are released into the solution, which cannot carry an electric current.
H2O | |
C12H22O11(s) | C12H22O11(aq) |
When two chlorine atoms come together to form a covalent bond, each atom contributes one electron to form a pair of electrons shared equally by the two atoms, as shown in the figure below. When a sodium atom combines with a chlorine atom to form an ionic bond, each atom still contributes one electron to form a pair of electrons, but this pair of electrons is not shared by the two atoms. The electrons spend most of their time on the chlorine atom.
Ionic and covalent bonds differ in the extent to which a pair of electrons is shared by the atoms that form the bond. When one of the atoms is much better at drawing electrons toward itself than the other, the bond is ionic. When the atoms are approximately equal in their ability to draw electrons toward themselves, the atoms share the pair of electrons more or less equally, and the bond is covalent. As a rule of thumb, metals often react with nonmetals to form ionic compounds or salts, and nonmetals combine with other nonmetals to form covalent compounds. This rule of thumb is useful, but it is also naive, for two reasons.
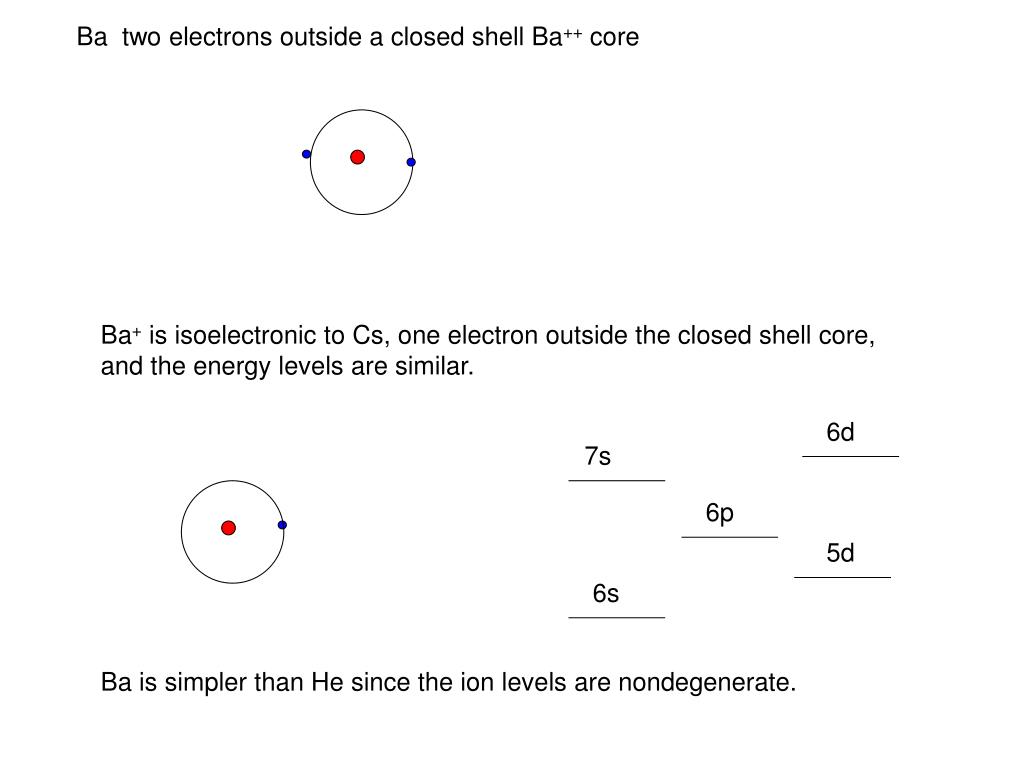
- The only way to tell whether a compound is ionic or covalent is to measure the relative ability of the atoms to draw electrons in a bond toward themselves.
- Any attempt to divide compounds into just two classes (ionic and covalent) is doomed to failure because the bonding in many compounds falls between these two extremes.
The first limitation is the basis of the concept of electronegativity. The second serves as the basis for the concept of polarity.
The relative ability of an atom to draw electrons in a bond toward itself is called the electronegativity of the atom. Atoms with large electronegativities (such as F and O) attract the electrons in a bond better than those that have small electronegativities (such as Na and Mg). The electronegativities of the main group elements are given in the figure below.
When the magnitude of the electronegativities of the main group elements is added to the periodic table as a third axis, we get the results shown in the figure below.
There are several clear patterns in the data in the above two figures.
- Electronegativity increases in a regular fashion from left to right across a row of the periodic table.
- Electronegativity decreases down a column of the periodic table.
When the difference between the electronegativities of the elements in a compound is relatively large, the compound is best classified as ionic.
Example: NaCl, LiF, and SrBr2 are good examples of ionic compounds. In each case, the electronegativity of the nonmetal is at least two units larger than that of the metal.
NaCl | LiF | SrBr2 | |||
Cl | EN = 3.16 | F | EN = 3.98 | Br | EN = 2.96 |
Na | EN = 0.93 | Li | EN = 0.98 | Sr | EN = 0.95 |
EN = 2.23 | EN = 3.00 | EN = 2.01 |
We can therefore assume a net transfer of electrons from the metal to the nonmetal to form positive and negative ions and write the Lewis structures of these compounds as shown in in the figure below.
These compounds all have high melting points and boiling points, as might be expected for ionic compounds.
NaCl | LiF | SrBr2 | |
MP | 801oC | 846oC | 657oC |
BP | 1413oC | 1717oC | 2146oC |
They also dissolve in water to give aqueous solutions that conduct electricity, as would be expected.
When the electronegativities of the elements in a compound are about the same, the atoms share electrons, and the substance is covalent.
Example: Examples of of covalent compounds include methane (CH4), nitrogen dioxide (NO2), and sulfur dioxide (SO2).
CH4 | NO2 | SO2 | |||
C | EN = 2.55 | O | EN = 3.44 | O | EN = 3.44 |
H | EN = 2.20 | N | EN = 3.04 | S | EN = 2.58 |
EN = 0.35 | EN = 0.40 | EN = 0.86 |
These compounds have relatively low melting points and boiling points, as might be expected for covalent compounds, and they are all gases at room temperature.
CH4 | NO2 | SO2 | |
MP | -182.5oC | -163.6oC | -75.5oC |
BP | -161.5oC | -151.8oC | -10oC |
Inevitably, there must be compounds that fall between these extremes. For these compounds, the difference between the electronegativities of the elements is large enough to be significant, but not large enough to classify the compound as ionic. Consider water, for example.
Water is neither purely ionic nor purely covalent. It doesn't contain positive and negative ions, as indicated by the Lewis structure on the left in the figure below. But the electrons are not shared equally, as indicated by the Lewis structure on the right in this figure. Water is best described as a polar compound. One end, or pole, of the molecule has a partial positive charge (+), and the other end has a partial negative charge (-).
As a rule, when the difference between the electronegativities of two elements is less than 1.2, we assume that the bond between atoms of these elements is covalent. When the difference is larger than 1.8, the bond is assumed to be ionic. Compounds for which the electronegativity difference is between about 1.2 and 1.8 are best described as polar, or polar covalent.
Covalent: | EN | < 1.2 | |
Polar: | 1.2 < | EN | < 1.8 |
Ionic: | EN | > 1.8 |
Practice Problem 2: Use electronegativities to decide whether the following compounds are best described as either covalent, ionic, or polar. (a) Sodium cyanide (NaCN) (b) Tetraphosphorus decasulfide (P4S10) (c) Carbon monoxide (CO) (d) Silicon tetrachloride (SiCl4) |
Electronegativity summarizes the tendency of an element to gain, lose, or share electrons when it combines with another element. But there are limits to the success with which it can be applied. BF3 (EN = 1.94) and SiF4 (EN = 2.08), for example, have electronegativity differences that lead us to expect these compounds to behave as if they were ionic, but both compounds are covalent. They are both gases at room temperature, and their boiling points are -99.9oC and -86oC, respectively.
The source of this problem is that each element is assigned only one electronegativity value, which is used for all of its compounds. But fluorine is less electronegative when it bonds to semimetals (such as B or Si) or nonmetals (such as C) than when it bonds to metals (such as Na or Mg).
This problem surfaces once again when we look at elements that form compounds in more than one oxidation state. TiCl2 and MnO, for example, have many of the properties of ionic compounds. They are both solids at room temperature, and they have very high melting points, as expected for ionic compounds.
TiCl4 and Mn2O7, on the other hand, are both liquids at room temperature, with melting points below 0oC and relatively low boiling points, as might be expected for covalent compounds.
TiCl4 | Mn2O7 |
MP = -24.1oC | MP = -20oC |
BP = 136.4oC | BP = 25oC |

The principal difference between these compounds is the oxidation state of the metal. As the oxidation state of an atom becomes larger, so does its ability to draw electrons in a bond toward itself. In other words, titanium atoms in a +4 oxidation state and manganese atoms in a +7 oxidation state are more electronegative than titanium and manganese atoms in an oxidation state of +2.
As the oxidation state of the metal becomes larger, the difference between the electronegativities of the metal and the nonmetal with which it combines decreases. The bonds in the compounds these elements form therefore become less ionic (or more covalent).
The Difference Between Polar Bonds and Polar Molecules
The difference between the electronegativities of chlorine (EN = 3.16) and hydrogen (EN = 2.20) is large enough to assume that the bond in HCl is polar.
Because it contains only this one bond, the HCl molecule can also be described as polar.
The polarity of a molecule can be determined by measuring a quantity known as the dipole moment, which depends on two factors: (1) the magnitude of the separation of charge and (2) the distance between the negative and positive poles of the molecule. Dipole moments are reported is units of debye (d). The dipole moment for HCl is small: = 1.08 d. This can be understood by noting that the separation of charge in the HCl bond is relatively small (EN = 0.96) and that the H-Cl bond is relatively short.
C-Cl bonds (EN = 0.61) are not as polar as H-Cl bonds (EN = 0.96), but they are significantly longer. As a result, the dipole moment for CH3Cl is about the same as HCl: = 1.01 d. At first glance, we might expect a similar dipole moment for carbon tetrachloride (CCl4), which contains four polar C-Cl bonds. The dipole moment of CCl4, however, is 0. This can be understood by considering the structure of CCl4 shown in the figure below. The individual C-Cl bonds in this molecule are polar, but the four C-Cl dipoles cancel each other. Carbon tetrachloride therefore illustrates an important point: Not all molecules that contain polar bonds have a dipole moment.
In vacuum tubes and gas-filled tubes, a hot cathode or thermionic cathode is a cathode electrode which is heated to make it emit electrons due to thermionic emission. This is in contrast to a cold cathode, which does not have a heating element. The heating element is usually an electrical filament heated by a separate electric current passing through it. Hot cathodes typically achieve much higher power density than cold cathodes, emitting significantly more electrons from the same surface area. Cold cathodes rely on field electron emission or secondary electron emission from positive ion bombardment, and do not require heating. There are two types of hot cathode. In a directly heated cathode, the filament is the cathode and emits the electrons. In an indirectly heated cathode, the filament or heater heats a separate metal cathode electrode which emits the electrons.
From the 1920s to the 1960s, a wide variety of electronic devices used hot-cathode vacuum tubes. Today, hot cathodes are used as the source of electrons in fluorescent lamps, vacuum tubes, and the electron guns used in cathode ray tubes and laboratory equipment such as electron microscopes.
Description[edit]
A cathode electrode in a vacuum tube or other vacuum system is a metal surface which emits electrons into the evacuated space of the tube. Since the negatively charged electrons are attracted to the positive nuclei of the metal atoms, they normally stay inside the metal and require energy to leave it.[1] This energy is called the work function of the metal.[1] In a hot cathode, the cathode surface is induced to emit electrons by heating it with a filament, a thin wire of refractory metal like tungsten with current flowing through it.[1][2] The cathode is heated to a temperature that causes electrons to be 'boiled off' of its surface into the evacuated space in the tube, a process called thermionic emission.[1]
There are two types of hot cathodes:[1]
- Directly heated cathode
- In this type, the filament itself is the cathode and emits the electrons directly. Directly heated cathodes were used in the first vacuum tubes. Today, they are used in fluorescent tubes and most high-power transmitting vacuum tubes.
- Indirectly heated cathode
- In this type, the filament is not the cathode but rather heats a separate cathode consisting of a sheet metal cylinder surrounding the filament, and the cylinder emits electrons. Indirectly heated cathodes are used in most low power vacuum tubes. For example, in most vacuum tubes the cathode is a nickel tube, coated with metal oxides. It is heated by a tungsten filament inside it, and the heat from the filament causes the outside surface of the oxide coating to emit electrons.[2] The filament of an indirectly heated cathode is usually called the heater.
The main reason for using an indirectly heated cathode is to isolate the rest of the vacuum tube from the electric potential across the filament, allowing vacuum tubes to use alternating current to heat the filament. In a tube in which the filament itself is the cathode, the alternating electric field from the filament surface would affect the movement of the electrons and introduce hum into the tube output. It also allows the filaments in all the tubes in an electronic device to be tied together and supplied from the same current source, even though the cathodes they heat may be at different potentials.
Valence Electrons In Each Element
To improve electron emission, cathodes are usually treated with chemicals, compounds of metals with a low work function. These form a metal layer on the surface which emits more electrons. Treated cathodes require less surface area, lower temperatures and less power to supply the same cathode current. The untreated thoriated tungsten filaments used in early vacuum tubes (called 'bright emitters') had to be heated to 2500 °F (1400 °C), white-hot, to produce sufficient thermionic emission for use, while modern coated cathodes produce far more electrons at a given temperature, so they only have to be heated to 800–1100 °F (425–600 °C).[1][3]
Types[edit]
Oxide-coated cathodes[edit]
The most common type of indirectly heated cathode is the oxide-coated cathode, in which the nickel cathode surface has a coating of alkaline earth metal oxide to increase emission. One of the earliest materials used for this was barium oxide; it forms a monatomic layer of barium with an extremely low work function. More modern formulations utilize a mixture of barium oxide, strontium oxide and calcium oxide. Another standard formulation is barium oxide, calcium oxide, and aluminium oxide in a 5:3:2 ratio. Thorium oxide may be used as well. Oxide-coated cathodes operate at about 800-1000 °C, orange-hot. They are used in most small glass vacuum tubes, but are rarely used in high-power tubes because the coating is degraded by positive ions that bombard the cathode, accelerated by the high voltage on the tube.[4]
For manufacturing convenience, the oxide-coated cathodes are usually coated with carbonates, which are then converted to oxides by heating. The activation may be achieved by microwave heating, direct electric current heating, or electron bombardment while the tube is on the exhausting machine, until the production of gases ceases. The purity of cathode materials is crucial for tube lifetime.[5] The Ba content significantly increases on the surface layers of oxide cathodes down to several tens of nanometers in depth, after the cathode activation process.[6] The lifetime of oxide cathodes can be evaluated with a stretched exponential function.[7] The survivability of electron emission sources is significantly improved by high doping of high‐speed activator.[8]
Barium oxide reacts with traces of silicon in the underlying metal, forming barium silicate (Ba2SiO4) layer. This layer has high electrical resistance, especially under discontinuous current load, and acts as a resistor in series with the cathode. This is particularly undesirable for tubes used in computer applications, where they can stay without conducting current for extended periods of time.[9]
Barium also sublimates from the heated cathode, and deposits on nearby structures. For electron tubes, where the grid is subjected to high temperatures and barium contamination would facilitate electron emission from the grid itself, higher proportion of calcium is added to the coating mix (up to 20% of calcium carbonate).[9]
Be Valence Electrons Octet
Boride cathodes[edit]
Lanthanum hexaboride (LaB6) and cerium hexaboride (CeB6) are used as the coating of some high-current cathodes. Hexaborides show low work function, around 2.5 eV. They are also resistant to poisoning. Cerium boride cathodes show lower evaporation rate at 1700 K than lanthanum boride, but it becomes equal at 1850 K and higher. Cerium boride cathodes have one and a half times the lifetime of lanthanum boride, due to its higher resistance to carbon contamination. Boride cathodes are about ten times as 'bright' as the tungsten ones and have 10-15 times longer lifetime. They are used e.g. in electron microscopes, microwave tubes, electron lithography, electron beam welding, X-Ray tubes, and free electron lasers. However these materials tend to be expensive.
Other hexaborides can be employed as well; examples are calcium hexaboride, strontium hexaboride, barium hexaboride, yttrium hexaboride, gadolinium hexaboride, samarium hexaboride, and thorium hexaboride.
Thoriated filaments[edit]
A common type of directly heated cathode, used in most high power transmitting tubes, is the thoriated tungsten filament, discovered in 1914 and made practical by Irving Langmuir in 1923.[10] A small amount of thorium is added to the tungsten of the filament. The filament is heated white-hot, at about 2400 °C, and thorium atoms migrate to the surface of the filament and form the emissive layer. Heating the filament in a hydrocarbon atmosphere carburizes the surface and stabilizes the emissive layer. Thoriated filaments can have very long lifetimes and are resistant to the ion bombardment that occurs at high voltages, because fresh thorium continually diffuses to the surface, renewing the layer. They are used in nearly all high-power vacuum tubes for radio transmitters, and in some tubes for hi-fi amplifiers. Their lifetimes tend to be longer than those of oxide cathodes.[11]
Thorium alternatives[edit]
Due to concerns about thorium radioactivity and toxicity, efforts have been made to find alternatives. One of them is zirconiated tungsten, where zirconium dioxide is used instead of thorium dioxide. Other replacement materials are lanthanum(III) oxide, yttrium(III) oxide, cerium(IV) oxide, and their mixtures.[12]
Other materials[edit]
In addition to the listed oxides and borides, other materials can be used as well. Some examples are carbides and borides of transition metals, e.g. zirconium carbide, hafnium carbide, tantalum carbide, hafnium diboride, and their mixtures. Metals from groupsIIIB (scandium, yttrium, and some lanthanides, often gadolinium and samarium) and IVB (hafnium, zirconium, titanium) are usually chosen.[12]
In addition to tungsten, other refractory metals and alloys can be used, e.g. tantalum, molybdenum and rhenium and their alloys.
A barrier layer of other material can be placed between the base metal and the emission layer, to inhibit chemical reaction between these. The material has to be resistant to high temperatures, have high melting point and very low vapor pressure, and be electrically conductive. Materials used can be e.g. tantalum diboride, titanium diboride, zirconium diboride, niobium diboride, tantalum carbide, zirconium carbide, tantalum nitride, and zirconium nitride.[13]
Cathode heater[edit]
A cathode heater is a heated wire filament used to heat the cathode in a vacuum tube or cathode ray tube. The cathode element has to achieve the required temperature in order for these tubes to function properly. This is why older electronics often need some time to 'warm up' after being powered on; this phenomenon can still be observed in the cathode ray tubes of some modern televisions and computer monitors. The cathode heats to a temperature that causes electrons to be 'boiled out' of its surface into the evacuated space in the tube, a process called thermionic emission. The temperature required for modern oxide-coated cathodes is around 800–1,000 °C (1,470–1,830 °F).
The cathode is usually in the form of a long narrow sheet metal cylinder at the center of the tube. The heater consists of a fine wire or ribbon, made of a high resistance metal alloy like nichrome, similar to the heating element in a toaster but finer. It runs through the center of the cathode, often being coiled on tiny insulating supports or bent into hairpin-like shapes to give enough surface area to produce the required heat. Typical heaters have a ceramic coating on the wire. When it's bent sharply at the ends of the cathode sleeve, the wire is exposed.The ends of the wire are electrically connected to two of the several pins protruding from the end of the tube. When current passes through the wire it becomes red hot, and the radiated heat strikes the inside surface of the cathode, heating it. The red or orange glow seen coming from operating vacuum tubes is produced by the heater.
There is not much room in the cathode, and the cathode is often built with the heater wire touching it. The inside of the cathode is insulated by a coating of alumina (aluminum oxide). This is not a very good insulator at high temperatures, therefore tubes have a rating for maximum voltage between cathode and heater, usually only 200 to 300 V.
Heaters require a low voltage, high current source of power. Miniature receiving tubes for line-operated equipment use on the order of 0.5 to 4 watts for heater power; high power tubes such as rectifiers or output tubes use on the order of 10 to 20 watts, and broadcast transmitter tubes might need a kilowatt or more to heat the cathode.[14] The voltage required is usually 5 or 6 volts AC. This is supplied by a separate 'heater winding' on the device's power supply transformer that also supplies the higher voltages required by the tubes' plates and other electrodes. One approach used in transformerless line-operated radio and television receivers such as the All American Five is to connect all the tube heaters in series across the supply line. Since all the heaters are rated at the same current, they would share voltage according to their heater ratings.
Battery-operated radio sets used direct-current power for the heaters (commonly known as filaments), and tubes intended for battery sets were designed to use as little filament power as necessary, to economize on battery replacement. The final models of tube-equipped radio receivers were built with subminiature tubes using less than 50 mA for the heaters, but these types were developed at about the same time as transistors which replaced them.
Where leakage or stray fields from the heater circuit could potentially be coupled to the cathode, direct current is sometimes used for heater power. This eliminates a source of noise in sensitive audio or instrumentation circuits.
The majority of power required to operate low power tube equipment is consumed by the heaters. Transistors have no such power requirement, which is often a great advantage.
Failure modes[edit]
Ba Number Of Valence Electrons
The emissive layers on coated cathodes degrade slowly with time, and much more quickly when the cathode is overloaded with too high current. The result is weakened emission and diminished power of the tubes, or in CRTs diminished brightness.
The activated electrodes can be destroyed by contact with oxygen or other chemicals (e.g. aluminium, or silicates), either present as residual gases, entering the tube via leaks, or released by outgassing or migration from the construction elements. This results in diminished emissivity. This process is known as cathode poisoning. High-reliability tubes had to be developed for the early Whirlwind computer, with filaments free of traces of silicon.
Slow degradation of the emissive layer and sudden burning and interruption of the filament are two main failure modes of vacuum tubes.
Transmitting tube hot cathode characteristics[15][edit]
Material | Operating temperature | Emission efficacy | Specific emission |
---|---|---|---|
Tungsten | 2500 K() | 5 mA/W | 500 mA/cm2 |
Thoriated tungsten | 2000 K(1726c) | 100 mA/W | 5 A/cm2 |
Oxide coated | 1000 K | 500 mA/W | 10 A/cm2 |
Barium aluminate | 1300 K | 400 mA/W | 4 A/cm2 |
See also[edit]
References[edit]
- ^ abcdefAvadhanulu, M.N.; P.G. Kshirsagar (1992). A Textbook Of Engineering Physics For B.E., B.Sc. S. Chand. pp. 345–348. ISBN978-8121908177.
- ^ abFerris, Clifford 'Electron tube fundamentals' in Whitaker, Jerry C. (2013). The Electronics Handbook, 2nd Ed. CRC Press. pp. 354–356. ISBN978-1420036664.
- ^Jones, Martin Hartley (1995). A Practical Introduction to Electronic Circuits. UK: Cambridge Univ. Press. p. 49. ISBN978-0521478793.
- ^MA Electrode Requirements
- ^'Archived copy'. Archived from the original on 2006-02-05. Retrieved 2006-02-14.CS1 maint: archived copy as title (link)
- ^B. M. Weon; et al. (2003). 'Ba enhancement on the surface of oxide cathodes'. Journal of Vacuum Science and Technology B. 21 (5): 2184–2187. Bibcode:2003JVSTB..21.2184W. doi:10.1116/1.1612933.
- ^B. M. Weon and J. H. Je (2005). 'Stretched exponential degradation of oxide cathodes'. Applied Surface Science. 251 (1–4): 59–63. Bibcode:2005ApSS..251...59W. doi:10.1016/j.apsusc.2005.03.164.
- ^B. M. Weon; et al. (2005). 'Oxide cathodes for reliable electron sources'. Journal of Information Display. 6 (4): 35–39. doi:10.1080/15980316.2005.9651988.
- ^ abElectron Tube Design, Radio Corporation of America, 1962
- ^Turner page 7-37
- ^'Archived copy'. Archived from the original on 2006-04-08. Retrieved 2006-02-14.CS1 maint: archived copy as title (link)
- ^ abElectron emission materials and components: United States Patent 5911919
- ^Thermionic cathode: United States Patent 4137476
- ^Sōgo Okamura History of electron tubes, IOS Press, 1994 ISBN90-5199-145-2, pp. 106, 109, 120, 144, 174
- ^L.W. Turner,(ed), Electronics Engineer's Reference Book, 4th ed. Newnes-Butterworth, London 1976 ISBN0408001682 pg. 7-36
External links[edit]
Be Valence Electrons Number
- Lankshear, Peter (July 1996). 'Valve filament/heater voltages'(PDF). Electronics Australia. Retrieved 9 October 2017.
Be Valence Electrons
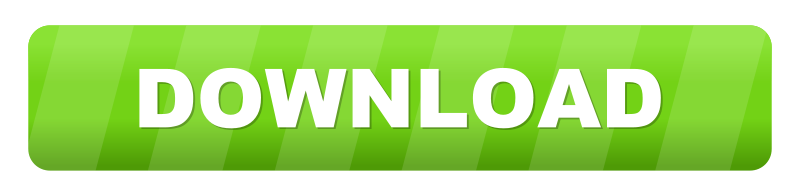